Featured Speakers

Metals usually exist in form of polycrystalline solids with crystalline grains separated by networks of grain boundaries (GBs) that are structurally disordered. The GBs are unstable and tend to get eliminated through grain coarsening upon heating or straining, or to transform into metastable amorphous states when the grains are extremely fine. This is why nano-grained metals have a much reduced stability relative to their coarse-grained counterparts, which is the major bottleneck limiting their processing and technological applications. Through experiments and MD simulations, we recently discovered a novel metastable state for extremely fine-grained metals (typically below 10 nm), namely Schwarz crystal structure. The GB-network of the metal is characterized by 3D minimal interfaces structure (with a zero-mean-curvature) constrained by twin boundaries. The unique structure is thermally stable against grain coarsening even close to the equilibrium melting point and exhibits a hardness in vicinity of the theoretical value. The across-boundary diffusion is so effectively suppressed that the diffusion-controlled processes such as intermetallic precipitation are inhibited. In this presentation, I will introduce the formation process, structure characteristics, and some properties of the Schwarz crystal structures in a number of pure metals and alloys.
References
1. X.Y. Li, Z.H. Jin, X. Zhou, K. Lu, Science, 370, 831-836 (2020).
2. W. Xu, B. Zhang, X.Y. Li, K. Lu, Science, 373, 683-687 (2021).
3. Z.H. Jin, X.Y. Li, K. Lu, PRL, 127, 136101 (2021).
Keynote Speakers

Advanced metallic materials with ultrahigh strength and large damage tolerance are urgently required in the field of aerospace, advanced transportation and nuclear energy where materials have to be exposed to most challenging environments, including extreme loading, temperatures and irradiations. However, simultaneously improving the strength and damage tolerance of the structural materialsis is extremely difficult due to the fact that the hardening media for increasing strength usually weakens the damage tolerance under various environments, especially in the ultrahigh-strength regime. In our work, a novel alloy design strategy was proposed to develop advanced high-performance metallic materials through the formation of coherent nanostructures, which interact with other crystal defects, such as dislocations, grain boundaries and irradiation defects, in an exceptional fashion. As a result, the long-standing tradeoff between the strength and damage tolerance was solved and a new grade of ultrahigh strength materials including steels and refractory high-entropy alloys with prominent mechanical properties and radiation tolerance were developed via tailoring the ordered nanostructures in different alloy matrices. The involved alloy design strategy and the underlying mechanisms responsible for the outstanding properties and unique performance behaviors will be discussed in detail.

High strength aluminium alloys are backbone structural materials for electrical vehicles, planes and spaceships. They must fulfil (at least) the following criteria: high strength, good formability, recyclability and mass producibility, and resistance to corrosion and hydrogen embrittlement[1].
The first two criteria require to design alloys with complex microstructures which introduces multiple local galvanic elements and undesired interfacial decoration phenomena that affect corrosion and hydrogen embrittlement. The sustainability criterion of making such alloys fully recyclable introduces the challenge of compositional variation and the intrusion of undesired tramp elements, both of which also influence the materials' behaviour in real environments[2].
In this presentation we discuss the specific influence of these features on hydrogen embrittlement. For this purpose, we performed atomic-scale investigations of hydrogen trapped in second-phase particles and at grain boundaries in a high-strength 7xxx Al alloy [3,4]. We used these observations to guide atomistic ab initio calculations, which show that the co-segregation of alloying elements and hydrogen favours grain boundary decohesion, and the strong partitioning of hydrogen into the second-phase particles removes solute hydrogen from the matrix, hence preventing hydrogen embrittlement. Our insights advance the mechanistic understanding of hydrogen-assisted embrittlement in Al alloys, emphasizing the role of hydrogen traps in minimizing cracking and guiding future hydrogen-resistant alloy design.
References
1. D. Raabe et al.: Making Sustainable Aluminum by Recycling Scrap: The Science of “Dirty” Alloys. Prog. Mater. Sci. 2022, 100947.
2. D. Raabe, D. et al.: Strategies for Improving the Sustainability of Structural Metals. Nature 2019, 575, pp 64–74.
3. H. Zhao et al.:Hydrogen Trapping and Embrittlement in High-Strength Al-Alloys. Nature 2022, 602, pp 437–441.
4. H. Zhao et al.: Interplay of Chemistry and Faceting at Grain Boundaries in a Model Al Alloy. Phys. Rev. Lett. 2020, 124 (10)

Vice President, Pathfinding for System Integration, TSMC
Semiconductor technology migration has followed Moore’s Law in chip scaling for around 60 years. Along those years, advanced materials have been introduced to enable chip (system-on-chip, SoC) scaling and provide performance, power efficiency and area/size values. When it becomes more challenging to continue Moore’s Law, wafer-level-system integration technologies, such as 2.5D and 3DIC have emerged to achieve system-on-package (SoP) scaling to enable system-level performance, power efficiency and size/volume values. System integration of chiplets is complementary to SoC scaling and support Moore’s Law extension. Advanced materials are also introduced in the packaging to realize the SoP scaling.
The implementation of new and advanced materials often introduces various kind of processing and integration challenges to be addressed. In this presentation, we will review some examples of introducing advanced materials in both SoC and SoP on their challenges and the approaches to resolve those issues. Looking forward, we would like to continue supply chain collaboration, innovation and leverage existing resources and capabilities to introduce more advanced materials to continue SoC- and SoP-Scaling.
Invited Speakers

1.Department of Materials Science and Engineering, National Yang Ming Chiao Tung University, Hsin-Chu, Taiwan 30010
2.Department of Materials Science and Engineering, National Chiao Tung University, Hsin-Chu, Taiwan 30010
Cu-to-Cu direct bonding has been implemented in high-end microelectronic devices with ultra-high input/output (I/O) density. The pitch of the Cu-Cu joints can be scaled below 1 µm, and thus its I/O density can be 300 times larger than that of the solder microbumps. In this presentation, the fundamental mechanism and interfacial microstructures of Cu-Cu direct bonding will be presented. A creep bonding model will be proposed. Then Cu/SiO2 hybrid bonding will be introduced. We adopted (111)-oriented Cu with high surface diffusivity to achieve low-temperature and low-pressure Cu/SiO2 hybrid bonding. The bonding temperature can be lowered from 300 °C to 200 °C, and the pressure is as low as 1.06 MPa. The bonding process can be accomplished by a 12-inch wafer-to-wafer or die-to-die scheme. The measured specific contact resistance is 1.2 x 10−9 Ω·cm2, which is the lowest value reported in literature for Cu-Cu joints bonded below 300 °C. The joints possess excellent electric properties up to 375 °C. Reliabilities of the Cu-Cu joints will be also presented.

Many electronic devices, such as field-effect transistors, depend on achieving precise control of both a semiconductor nanostructure and its contact with the larger scale circuit. The control of the contact between nanowire and circuit is a key step that involves integrating different types of materials and bridging between length scales. In Si nanowires, we show that silicide formation can occur through a point contact reaction and we demonstrate that the reaction shows different kinetics from those already known in thin film silicide technology. We discuss the strain effect on the nucleation and growth of silicides in nanowires with thermodynamic, kinetic, and strain energy implications. Such nanowires have an oxidized surface and this controls the reaction pathway and kinetics. To follow up the present model, the gradient of stress potential is treated as the driving force for “uphill diffusion” of metal atoms in Si to migrate to the epitaxial interface. Additionally, the strain effect is taken as a reason that an extremely high degree of supersaturation of Ni, over a factor of 1000 needed for NiSi formation, can take place near the interface. The need of an extremely high super-saturation, about a factor of 1000, of Ni interstitials for the nucleation is because of the extremely low equilibrium solubility of Ni in Si. Also what is the diameter of the point contact is irrelevant, provided that it is not closed to stop the reaction.
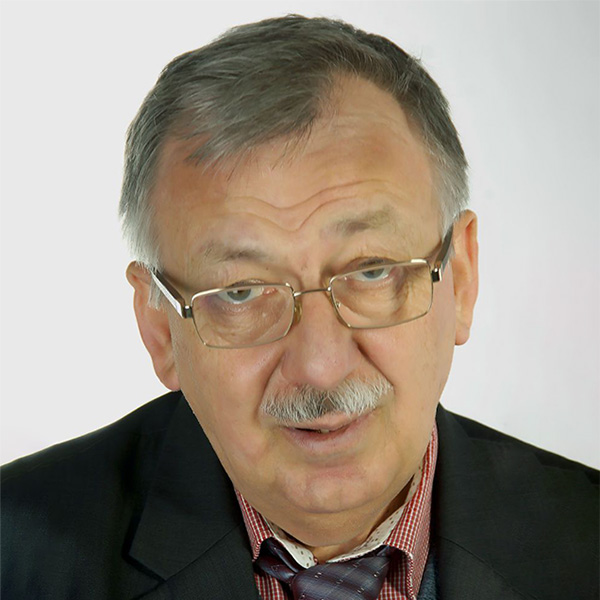
Failure of the microelectronic device may be treated in the frame of non-equilibrium thermodynamics. Three main ideas are discussed:
Failure may be treated as a result of accumulation of structural entropy beyond some threshold value. Accumulation of structural entropy is calculated as a "non-heating" part of entropy production in the processes of electromigration, thermomigration and stress migration. Such approach leads to the Black's equation for MTTF with exponent n=2 for electromigration-induced failure, and to analogous predictions for thermomigration-induced failure, as well as for stress migration-induced failure.
Failure may be treated as a kind of the first-order phase transformation in an open system, and includes nucleation, growth or migration and (sometimes) ripening. Moreover, the usually used cumulative Weibull distribution for time to failure (TTF) looks very similar to Kolmogorov-Avrami equation for crystallization kinetics describing nucleation and growth of crystalline phase[1]. Several examples of failure mechanisms are analyzed within this framework.
Failure may be treated as a result of incompatibility of steady-states for different processes in an open system. For example, steady-state current under fixed voltage between the cathode and anode ends of interconnect leads to electromigration of atoms and vacancies which, in realistic materials, lead to non-steady-state voiding and hillock formation, and to eventual failure. Fully compatible steady-states for all processes would mean "immortality" at least for interconnect.
References
[1] TU, King-Ning. Electronic thin-film reliability. Cambridge University Press, 2010.

High strength steels are widely used in various industries. Understanding the strain hardening mechanism of high strength steel plays a key role on the development of new class of high strength steel. The first part of the present work revisit the twinning-induced plasticity (TWIP) effect on the strain hardening mechanism of TWIP steel. It is found that TWIP effect has trivial effect on the strain hardening of TWIP steel. Instead, carbon-induced high dislocation in TWIP steel is the major mechanism responsible for the high strain hardening of TWIP steels. The second part revisit the TRIP effect on strain hardening of TRIP-assisted steels at high-strain-rate deformation. During high-strain-rate deformation, martensitic transformation does occur, but the strain hardening rate is still low, indicating that TRIP effect does not provide strain hardening behaviour at high-strain rate. Further investigation indicates that the reason for this abnormal TRIP effect at high strain rate could be attribute to the critical role of interstitial carbon played in the TRIP effect.

Nano-lamellar materials with ultrahigh strengths and unique physical properties are of technological importance for structural applications. However, these materials generally suffer from low tensile ductility, which severely limits their practical utility. In this talk, we show that markedly enhanced tensile ductility can be achieved in coherent nano-lamellar high-entropy alloys, which exhibit an unprecedented combination of over 2 GPa yield strength and 16% uniform tensile ductility. The ultrahigh strength originates mainly from the lamellar boundary strengthening, whereas the large ductility correlates to a progressive work-hardening mechanism regulated by the unique nano-lamellar architecture. The coherent lamellar boundaries facilitate the dislocation transmission, which eliminates the stress concentrations at the boundaries. Meanwhile, deformation-induced hierarchical stacking-fault networks and associated high-density Lomer-Cottrell locks enhance the work hardening response, leading to unusually large tensile ductilities. The coherent nano-lamellar strategy can potentially be applied to other alloys and open new avenues for designing ultrastrong-yet-ductile materials for technological applications.

Recently, exceptional properties that are continuously found in an intriguing new class of metallic structural materials, high-entropy alloys (HEAs), demonstrate their great potential for engineering applications particularly in extreme environments where conventional alloys reach their limits. The concept of HEAs has revolutionized traditional alloy design, in particular in terms of their endless composition space, which meanwhile brings a new challenge of how to effectively design HEAs with targeted properties. In this presentation, we will give an introduction on HEAs first. Following that, we will focus on the design of advanced precipitate-strengthened lightweight HEAs for high-temperature applications by integrating computational methods and advanced experimental techniques, such as neutron scattering. We will discuss how to design high-strength, low-cost, and lightweight HEAs by the Calculation of Phase Diagrams (CALPHAD)-based high-throughput computational method (HTCM). A fundamental understanding of the precipitation-strengthening and order-disorder-transition behaviors in these newly-designed lightweight HEAs is revealed by in-situ neutron scattering, advanced microscopies, Monte-Carlo (MC) simulations, and ab initio molecular dynamics (AIMD). This study provides in-depth insights into the discovery of advanced structural materials by the HEA concept.
*R. Feng, C. Zhang, M.C. Gao, Z. Pei, F. Zhang, Y. Chen, D. Ma, K. An, J.D. Poplawsky, L. Ouyang, Y. Ren, J.A. Hawk, M. Widom, P.K. Liaw, High-throughput design of high-performance lightweight high-entropy alloys, Nature Communications 12(1) (2021) 4329.

The pandemic of coronavirus SARS-COV-2 leads us to the question of how to make human society more reliable under the strike of virus. The virus with a high spontaneous mutation rate challenges the cognitive process and the development of antiviral agents. The killing of virus and bacteria on copper surfaces is known, and the mechanism is explained as copper ions released from a copper surface. On an ordinary copper surface, it will take a few hours to kill the virus. In this talk, we try to enhance the virus-killing reaction rate by the design of copper surface microstructure. We made a copper surface by depositing (111)–oriented nanotwin and studied the killing effect of Feline Infectious Peritonitis Virus (FIPV). By making clear the chemistry and physics mechanism behind virus killing on copper surfaces, we will try to increase the virus-killing rate further. In the future, we can make a copper filter for air quality control in public buildings, for example, the filter can be installed in the ventilation system, on the ceiling of restaurants, or in other common places to purify the air and prevent the spread of the contaminated air. We can also make recyclable copper masks for individual people.

Metal-metal composite (MMC) is a typical heterogeneous material that consists of metal matrix and secondary metallic phase. Due to the compositional gradient interface, MMCs usually have a better combination of strength and ductility compared with conventional ceramic reinforced composites. However, the effecting mechanism of gradient interface on mechanical behavior of MMCs is still unclear. In this study, we proposed a powder metallurgical method to prepare titanium matrix MMCs, such as Ti-Ta, Ti-Mo and Ti-W composites. A superior combination of tensile strength (1200~1500 MPa) and elongation (8~20%) was achieved. It was found that the structural gradient interface, produced by the compositional gradient, could generate strong hetero-deformation induced (HDI) stress, which consequently enhanced the strength of the MMCs. Meanwhile, multiple deformation modes including dislocation planar sliding, stress-induced twining (TWIP), and stress-induced martensite phase transformation (TRIP) were activated around the gradient interface during plastic deformation, which help to release stress concentration and increase strain hardening capability, thereby resulting in a good ductility. Moreover,the mechanical Behavior of Ti-metal composites under high strain rates were also reported. Besides, we have proposed a new interface stress model to evaluate the HDI stress from the perspective of residual strain energy. The HDI stress values obtained from the new interface stress model can more reasonably explain the interfacial strengthening effects than those from the well-used back stress model for heterogeneous-structured materials.
Keywords: Metal-metal composites; Strengthening mechanis; Interfacial stress model

Blocking of viruses at the portal of entry is an extremely attractive approach to combat infections and for pandemic preparedness. We have explored various strategies for the inhibition of viruses at mucosal surfaces, particularly in the oronasal cavity. Our work has focused on carbon-based architectures, especially graphene and its derivatives. One of the major problems in the development of novel virus inhibitor systems is the adaption of the inhibitor to the size of virus particles, and we synthesized and tested carbon-based inhibitors of different sizes to evaluate potential size effects on the inhibition of virus entry and replication. Nanomaterials were functionalized with polygylcerol through a "grafting from" polymerization to form new polyvalent nanoarchitectures, which allowed polysulfation to mimic the heparan sulfates present on cell surfaces that we reasoned would compete with the binding sites of herpes viruses. Inhibitory efficiency is regulated by the size of the polymeric nanomaterials and the degree of sulfation and the derivatives inhibited virus infection at an early stage as predicted. In similar work, inhibition of SARS-CoV-2 by graphene platforms with precise dual sulfate/alkyl functionalities was investigated. A series of graphene derivatives with different lengths of aliphatic chains were synthesized and we showed that graphene derivatives with long alkyl chains (>C9) inhibit coronavirus replication by virtue of disrupting viral envelope.

SARS-CoV-2, a novel coronavirus, was emerged in humans in 2019. The virus has been quickly transmitted around the world in weeks and led to the declaration of COVID-19 pandemic by WHO in March 2020. In the initial stage of the COVID-19 outbreak, there was very limited understanding about the novel pathogen. Epidemiological studies indicate that COVID-19 majorly spread via the droplet transmission route between people in close contact. However, the disease is also possible to transmit via the indirect contact route, i.e. a person becomes infected when touching their eye, nose or mouth after touching a virus-contaminated surface or object. In addition, there are evidences that facilities occupied by COVID-19 patients are heavily contaminated with SARS-CoV-2. In order to better understand the modes of transmission of COVID-19 in human populations, knowledge related to physical or environmental parameters that can control the stability and transmissibility of SARS-CoV-2 is warranted. In this seminar, we will discuss the stability of SARS-CoV-2 in different environmental conditions. The effects of commonly used disinfectants on the virus and protocols for disinfecting virus-contaminated surfaces will also be studied. In addition, we will also discuss the virucidal effects of different metallic surfaces. These findings can help to develop approaches for inactivating SARS-CoV-2 in environmental surfaces, thereby reducing the risk of fomite transmission.
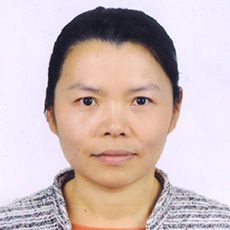
Three-dimension (3D) integration with TSV technology has been regarded as a promising way to overcome the limitation of Moore’s law. The conventional physical vapor deposition (PVD) has shown difficulty in depositing the conformal barrier/liner and Cu seed layer in high aspect ratio (HAR) TSV. Other alternative methods such as chemical vapor deposition (CVD) and atomic layer deposition (ALD) are both based on the high-temperature and high-cost process to obtain the conformal film. Electroless deposition (ELD) technology is a robust candidate to replace the PVD in the HAR TSV metallization owing to its advantages of conformal film coverage and low cost. Co has been considered as an excellent liner material because of its better wettability with Cu and lower resistivity than Ta. The application of the ELD pure Co layer in TSV has not been reported. In this presentation, we demonstrated a wet processes flow for HAR TSV metallization. The ELD Co liner and alkaline electroplating deposited Cu seed layer are successfully integrated into a 4 μm×50 μm TSV. The ELD Co film can have a low resistivity of 13.6 μΩ·cm, due to high metallic Co purity of about 99.4%. The Co liner layer with a step coverage of up to 98% is formed on the TiN barrier in the TSV followed by a conformal alkaline Cu seed layer with step coverage of 75%. Finally, the TSV is filled without voids by the acidic Cu electroplating. This work demonstrates the feasibility of the wet processes flow of conformal liner and seed layer in the HAR TSV.

Low entropy means low waste heat, for processes which use low temperature and low electrical current density. In the beginning, the Centre will focus on reliability issues in 3D IC due to Joule heating as well as heat dissipation. Specifically, electromigration, thermo-migration, and stress-migration failures will be analysed. Three examples are given below. First, based on Onsager’s theoretical study of entropy production, we can perform 1T1j (one temperature and one current density) rather than 3T3j to obtain the statistical distribution of mean-time-to-failure of a device. Furthermore, we can calculate Imax. Second, we developed experimentally the room temperature solid-state solder joint formation. It is different from the conventional solid-liquid interfacial diffusion (SLID) based solder joint formation at 250 ℃. Third, we perform low temperature Cu-to-Cu and hybrid bonding. In the long run, the link between semiconductor technology and bio-medical applications will be emphasized. Our cut-in point is to use Cu cloth and (111) oriented nano-twin Cu to inactivate COVID-19 virus.

High entropy alloys have been recently attracted tremendous research interest worldwide because of their excellent mechanical, chemical and physical properties. In this talk, I would like to discuss our recent work that aims to address some fundamental questions in this field, such as entropic design of alloys and lattice distortion. Through extensive experiments and simulations, we are able to show that it is possible to develop multi-functional high entropy alloys by carefully mixing elements of distinct sizes, which could exhibit a combination of unique mechanical/physical properties, such as superb strength, excellent elastic strain limit, ultralow mechanical hysteresis, remarkable elinvar effect, superior plasticity and many others. At the fundamental level, we found that the multi-functionality of these high order alloys could be attributed to not only chemical fluctuations, but also the presence of heterogeneous lattice strains, both of which however are lacking in conventional alloys.

Intermetallic materials are bestowed by diverse ordered superlattice structures together with many unusual properties. In particular, the advent of chemically complex intermetallic alloys (CCIMAs) has received considerable attention in recent years and offers a new paradigm to develop novel metallic materials for advanced structural applications. These newly emerged CCIMAs exhibit synergistic modulations of structural and chemical features, such as self-assembled long-range close-packed ordering, complex sublattice occupancy, and interfacial disordered nanoscale layer, potentially allowing for superb physical and mechanical properties that are unmatched in conventional metallic materials. In this talk, we would like to introduce the historical developments and recent advances in ordered intermetallic materials from the simple binary to chemically complex alloy systems. We are focused on the unique multicomponent superlattice microstructures, nanoscale grain-boundary segregation and disordering, as well as the various extraordinary mechanical properties of these newly developed CCIMAs. Finally, perspectives on the future research orientation of this new frontier are presented.
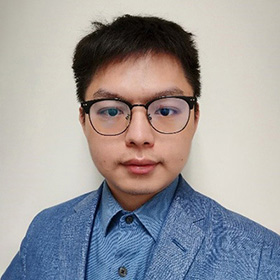
The prevalence of COVID-19 has caused global dysfunction in public health, sustainability, and socio-economy. While vaccination shows potential in containing the spread, the virus variants and co-infection with other diseases such as fatal fungal infections and monkeypox have further exacerbated the scenario. Therefore, the development of surfaces showing broad-spectrum antiviral and antimicrobial activities is imperative, especially amid the early stage of an unknown pandemic.
In this presentation, we will introduce our recent development of laser-induced graphene (LIG) technology for fighting against disease transmission. We will start with the introduction of LIG, a revolutionary technique to synthesize porous graphene film from universal carbon precursors. Then we will focus on our strategies for surface engineering of LIG, which enable rapid and efficient disinfection under mild conditions. The intrinsic, photothermally and electrically enhanced antimicrobial and antiviral activities of different types of LIG will be discussed. Lastly, we will showcase other developments of the LIG techniques and highlight their impacts on the environment, including surface sensing via hygroelectricity, degradation via Joule-heating, and water disinfection via electrochemistry.

“High-Entropy Materials (HEMs)” has become an emerging field through the collective efforts of many researchers in the last two decades. Such materials were revolutionary because they were historically thought to be difficult or even impossible to be fabricated and applicable due to their brittleness by nature. High entropy effect which was ignored before has become well-known to enhance the formation of multielement solution phases and avoid complicated microstructure with complex phases. As the long-standing bottlenecks of conventional materials were difficult to be solved by conventional materials concept, HEMs thinking will increase the whole materials ability to solve the conventional bottlenecks. Various categories of materials with high entropy effect have been developed, which indeed display promising properties for different applications. It is the time to build HEMs technology which could transfer those promising discoveries into industrial production and thus create well-being for our society. In this presentation, the main bottlenecks in conventional materials and the ESG issues in energy, waste, pollution, natural resource conservation are pointed out to emphasize the large opportunities of HEMs and related materials. Some industrialized examples of high-entropy materials technology are also presented.